This is the second article of a series on the MakAir open-source ventilator, a project that I founded with friends, which was born at the beginning of the COVID-19 pandemic in early 2020. Think of this series of articles as a ledger of all that happened and all that was created. These articles could serve as the foundation of a project reboot in future years, in the event a similar pandemic was to emerge again.
This article contains the medical knowledge that’s required to understand how mechanical ventilation works. It takes a problem-first approach, as we want to avoid any complex theory there, which would be useless to the engineers we are. This article will thus explain in a simple way how to (medically) ventilate a patient, as well as teach some important medical vocabulary. This vocabulary will be re-used in further articles when explaining how things work on the engineering side. Let’s dive into it!
👉🏻 Disclaimer: I am not a doctor, nor do I have any background in the medical field. I am an engineer by training. This content should be considered for engineering purposes only, as it might be sometimes imprecise, though sufficient for building a ventilator.
How Human Lungs Work
Volume and pressure basics
A typical human breathes 12 times per minute, and circulates around 6L of air in and out of their lungs, at rest. That’s about 500mL of air per inspiration that goes in and out of the lungs, from which gas exchanges occur (O2 enters the system, CO2 leaves it).
That’s about volume. Speaking about the pressure of air inside the lungs during a respiratory cycle, and showing it as a graph as a function of time, we get:
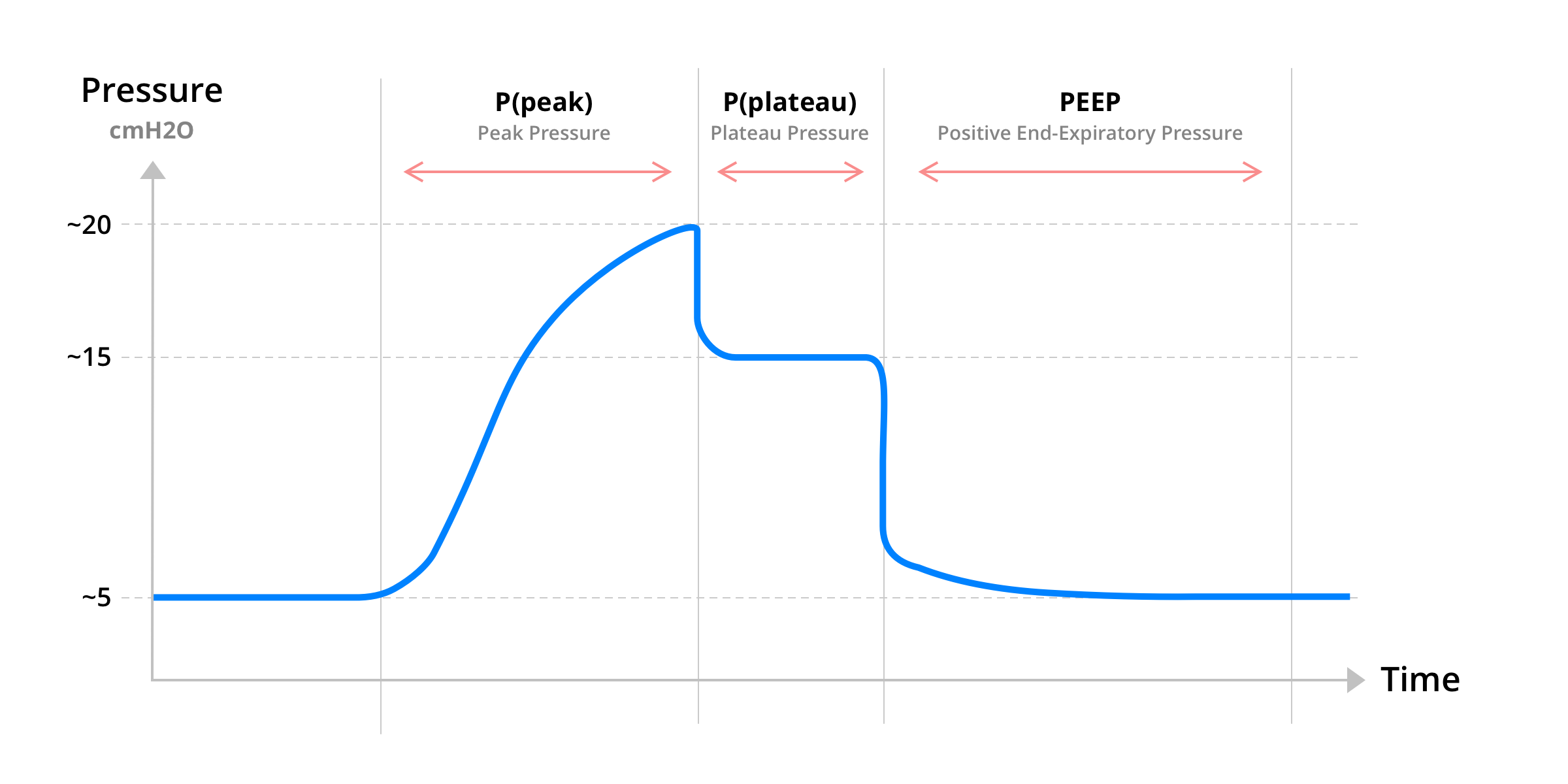
As you can see on the graph above, the pressure in the lungs can be split in 3 phases for a full respiratory cycle. That’s peak pressure: P(peak)
, plateau pressure: P(plateau)
and positive end-expiratory pressure: PEEP
, also called P(expiratory)
.
The lung respiratory cycle can be summed up as such, in terms of pressure:
- The lungs inspire air in: peak pressure occurs;
- The lung resistance blows a bit of air out: the pressure stabilizes, coming to a plateau;
- The diaphragm relaxes and the lungs expire air out: pressure decrease, though retaining a minimum air pressure inside (the positive end-expiratory pressure).
Note that this pressure cycle is super important to understand, in order to continue with the aspects of mechanical ventilation.
Forcing a given pressure for each stage of the graph above ensures a certain volume of air gets in the lungs. This volume of air contains a certain amount of O2, hence why the pressure needs to be tuned in order for the required amount of O2 to be provided.
As well, the lungs can only accept so much pressure. During a natural ventilation cycle, pressure is under control by natural processes and the lungs are safe from any over-pressure event. During mechanical ventilation, over-pressure is a thing and should be closely monitored, as the ventilator could push too much air in the lungs, creating too much pressure inside of them.
The lungs alveoli are very fragile: while they can allow for short pressure spikes, sustaining an elevated pressure might permanently alter some of them, leaving long-term sequels on the patient’s respiratory capacity (ie. amount of gas exchange that can occur per breath).
Important ventilation concepts
At each breath, the lungs inflate with a given amount of air. This is the Tidal Volume, which can be described as the normal volume of air displaced between inhalation and exhalation, without applying extra effort. In a regular healthy person, tidal volume is around 500 mL per breath. It can also be expressed as a function of body mass, with 12 mL per kilogram of lean body weight at 12 cycles per minute. Calculating the tidal volume from body mass is handy when it comes to ventilating a patient, as each patient physiology has different oxygenation needs.
The air contains a stable percentage of oxygen at any altitude, that is 21%. The air pressure may vary, thus the total actual amount of O2 molecules vary as well, though the percentage of O2 in the air stays stable at all elevations. Whenever inspiring such natural air, the Fraction of Inspired Oxygen, a.k.a. FiO2, is 0.21
(21% O2). While ventilating a patient which experience difficulty breathing, the FiO2 value can be adjusted by inserting oxygen into the system, giving out a FiO2 between 0.21
and 0.5
. The FiO2 value could even go up to 1.0
for some conditions, that is, 100% of oxygen in the air. Note that when we refer to FiO2 values, the precision is always imperfect. When ventilating a patient, 100% O2 could be in reality 80% O2 due to physic constraints in the air flow. This would still be sufficient though to maintain the patient in a stable condition.
When lungs are inflating, an important metric that tells how easily air can get inside of them is the Lung Compliance, or pulmonary compliance. It is a measure of the lung’s ability to stretch and expand. We will mostly be referring to static lung compliance through this series of articles. Static lung compliance is the change of volume (in the lungs), for a given applied pressure (of air to the lungs). In other words, it tells how easily the lungs inflate when given input air.
At any given time, Oxygen Saturation in the blood can be measured. Oxygen saturation tells how well the lungs perform at their function, that is taking O2 in and giving back CO2 out. Oxygen saturation is the fraction of oxygen-saturated hemoglobin relative to total hemoglobin (unsaturated + saturated hemoglobin). Normal arterial blood oxygen saturation level is between 95% and 100%. Whenever this level goes below 90%, the body can get into a lot of trouble, ie. organs can fail and cardiac arrest can happen. Blood oxygen saturation is an important metric for hospitals to measure when ventilating a patient, using eg. pulse oximetry (SpO2). This tells about how efficient the ventilator is — and its configured settings — at keeping the patient well and alive.
What Happens For COVID-19 & ARDS Patients
Patients who develop Acute respiratory distress syndrome (ARDS) due to COVID-19, fail to maintain proper natural ventilation (oxygenation), due to a rapidly-evolving inflammation in the lungs. ARDS impairs the lungs ability to exchange O2 and CO2, which in turn pose a life-threatening risk on organs and heart.
People that once experienced ARDS exhibit long-term damage to their lungs function, for which respiratory capacity is dramatically decreased post-remission, affecting their quality of life.
While the underlying causes of ARDS are being treated, an ARDS patient might need to be kept stable under mechanical ventilation, depending on the situation. Mechanical ventilation settings are chosen based on the medical condition of the patient. Light conditions could be treated with non-invasive ventilation, using a simple face mask (the patient would be kept conscious). In worst cases, the patient would be put asleep, natural lung function would be deactivated, the patient would be intubated and ventilated in a (positive) pressure controlled mode.
Given that COVID-19 patients under invasive ventilation may stay intubated for 2 weeks, specific ventilators capable of handling prolongated ventilation need to be used. Not all ventilators are fit for this, as the most occurring ones are support ventilators used for ventilation aid, or emergency purposes (eg. in emergency vehicles). Only the bulkiest and most expensive ICU ventilators are capable of handling COVID-19 patients, for which the price can range from 25,000 USD to 50,000 USD (!!). Hamilton Medical and Dräger manufacture such ventilators.
How To Ventilate a Patient
Ventilation modes
Depending on the condition of the patient, the way they get ventilated can be chosen amongst a known list of ventilation modes.
Ventilation modes are named using acronyms, eg. PC-CMV
, where the first part of the acronym contains the mode (eg. PC
), and the second part tells about the mode behavior (eg. CMV
).
The most popular ventilation modes are Pressure Control (PC) and Volume Control (VC). Those are two schools of thought in ventilation, that are quite equivalent in terms of treatment outcomes. Medical personnel are usually more familiar with one or the other, which explains why they chose pressure over volume or volume over pressure.
For each ventilation mode, a specific behavior can be chosen. The respiratory cycles can be either forced by the ventilator, or requested by the patient. In the latter case, the ventilator would detect an inspiration trigger by the patient by sensing a drop in pressure, as the lungs would try to inflate. Some middle grounds exist, where the chosen ventilation mode would force a given number of respiratory cycles, while the patient could still ask spontaneously for a cycle between two forced cycles. What matters in that case is that the measured amount of cycles in the current minute approximates the configured cycles target.
As of end 2020, the MakAir ventilator implements 5 combinations of ventilation modes (3 pressure modes, 2 volume modes). Those are the most common ventilation modes, which can also be found on traditional ventilators.
Other more advanced, exotic or experimental ventilation modes exist, but we chose not to implement them. For instance, dual-control modes of ventilation exist, where the ventilator would try to reach both a target pressure and volume at the same time. For instance, with PRVC
or Pressure Regulated Volume Control, a tidal volume target is added to a Pressure Assist Control mode (PC-AC
).
Supported ventilation modes on the MakAir are:
Pressure CMV
or Pressure Continuous Mandatory Ventilation: respiratory rate is forced and a target pressure is set to be matched (note that this mode was the only one we supported on MakAir Model 1 in April 2020) (forced mode, invasive);Pressure AC
or Pressure Assist Control: a target respiratory rate and pressure are set, though the patient can trigger a cycle by inspiring air (forced mode with trigger, invasive);Pressure VSAI/BIPAP
or Bilevel Positive Airway Pressure: an inspiratory airway pressure is delivered (IPAP
), that is higher to the expiratory airway pressure (EPAP
), while the patient is kept fully responsive of triggering cycles. This mode is common in the treatment of sleep apnea (note thatVSAI
is a French term that we use, for whichBIPAP
is the English equivalent) (spontaneous mode, non-invasive);Volume CMV
or Volume Continuous Mandatory Ventilation: respiratory rate is forced, a target tidal volume is set to be matched (resulting in a target minute volume in the lungs, in L/min) (forced mode, invasive);Volume AC
or Volume Assist Control: a target respiratory rate and tidal volume are set, though the patient can trigger a cycle by inspiring air (forced mode with trigger, invasive);

Units in use
In the world of mechanical ventilation, some unusual units are used to express physical values such as pressure.
Most units in use on ventilators are time-based units, as well as fluid characterization units (pressure, volume, flow).
Units that are used in mechanical ventilation:
cmH2O
&mmH2O
: centimetre & millimetre of water, unit of pressure used to measure air pressure;ms
&s
: unit of time used eg. to calculate the inspiration-expiration ratio;L
&mL
: unit of volume, used eg. to express the volume of air in the lungs (the tidal volume);L/min
: unit of flow, used eg. to express the amount of air going in and out of the lungs every unit of time (the minute volume);cycles/minute
: number of respiratory cycles per minute;
Ventilation settings
Aside from configuring the ventilation mode, which defines the behavior of algorithms with measurements, ventilation target values can be configured as well. The ventilator will attempt to reach those target values at any time during ventilation (or ring an alert if it fails to do so).
Settings should be configured using educated doctor decisions & calculations, as every patient condition require specific tunings before they can be connected to the ventilator.
For instance, severe ARDS patients tend to have very resistive lungs that would collapse on themselves if high pressure could not be sustained in the lungs anytime, making it very hard to inflate again. This is known as the fibrotic stage of ARDS. In such cases, a doctor would make the decision to set an higher-than-usual PEEP
target.
Some settings are commonly used in mechanical ventilation:
P(peak)
in cmH2O: target peak pressure during inspiration (automatically calculated);P(plateau)
in cmH2O: target plateau pressure during inspiration;PEEP
orP(expiratory)
in cmH2O: target positive end-expiratory pressure, which is the minimum pressure held in the lungs after expiration;Inspiratory duration
in ms: target inspiratory duration;Inspiration-expiration ratio
as (1:x): target inspiratory-to-expiratory ratio;Tidal volume
in mL: volume of air pushed to the lungs at each cycle;Cycles per minute
in cycles/minute: number of breaths per minute (full respiratory cycle);Minute volume
in L/min: volume of air pushed to the lungs every minute (in other words:minute volume = (tidal volume x cycles per minute)
);
More advanced settings are available:
Trigger offset
in cmH2O: the drop in pressure that would trigger an inspiration (typically as low as a pressure offset of 2-5 cmH2O);Plateau duration
in ms: the duration of the plateau stage on the pressure curve (please refer to the typical pressure graph shown at the beginning of the article to visualize when plateau occurs);

Safety & alarms
For patient safety and regulatory compliance reasons, any mechanical ventilator must alert the medical personnel whenever an anomaly occurs.
Alarms must be loud and audible, and emit a specific pitch. The screen must also show the list of ongoing alarms, with different priorities, from low to high.
Most alarms are predefined and thus cannot be customized. For instance, the ventilator must be able to detect whenever the patient is disconnected from the ventilator. Some alarms can also be customized, depending on the current ventilation mode.
Custom alarms can be set up in order to alert medical teams when a value goes over or under a set threshold:
Inspiratory minute volume
in L/min: minimum and maximum allowed air flow per minute during inspiratory cycles;Expiratory minute volume
in L/min: minimum and maximum allowed air flow per minute during expiratory cycles;Respiratory rate
in cycles/minute: breathing cycles per minute that must be achieved;Tidal volume
in mL: volume that goes in and out of the lungs for each respiratory cycle (this one is important, as when multiplied with the number of cycles per minute, it tells how much O2 the patient takes in per minute);Leak volume
in mL/min: maximum volume of air per minute that is allowed to leak out of the circuit (any ventilation circuit has some level of leak, which can be due to the breathing system and/or the patient’s physiology);Peak pressure
in cmH2O: maximum allowed pressure during the peak stage of the pressure curve;

Closing Notes
This article was the second of a series on the MakAir story, providing insider details on the project, medical knowledge on the state of the art of mechanical ventilation, as well as in-depth engineering explanations on how we designed our ventilator algorithms and electronics. The overall goal of this series of articles is to build the legacy of the MakAir project.
Being an engineer and having no medical background, I would like to re-emphasis that this article is aimed at engineers and might contain medical imprecisions. If you are an engineer looking to understand how mechanical ventilation works by taking a first principle approach, this article might have helped you (please let me know!). If you are a doctor and you spotted errors, please leave a comment or contact me so that I can fix them!
All the medical knowledge contained in this article has been learned from online resources and volunteering doctors coming on-site during the time of the project to teach us medical concepts on a drawing board (literally!). We came from having zero knowledge on how the lungs work to what got us to design a working ventilator. We are still learning though, thanks to clinical trial results and further research related to adding new features and improving ventilation algorithms.
Also, I’m always available to answer questions, please ask them in the comments section of this article!
🇮🇹 Written from Venice, Italy.